Power Factor Improvement Panel
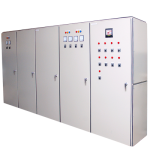
Key Features
General Description:
To obtain the best possible economic advantage from electric power both the generating plant and consumers plants should be operated at high efficiency. To achieve this it is essential to have a high power factor throughout the system.
Most a.c. electric machines draw from the supply apparent power in terms of kilovolt-amperes (KVA) which is in excess of the useful power measured in kilowatts(KW) required by the machine. The ratio of these quantities
Useful power/Apparent power or KW/KVA =Power factor (cos φ)
is known as the power factor of the load and is dependent upon the type of machine in use. A large proportion of the electric machinery used in industry has an inherently low power factor, which means that the supply authorities have to generate much more current than is theoretically required. In addition, transformers and cables have to carry this extra current. When the overall power factor of generating station’s load is low, the system is inefficient and the cost of electricity correspondingly high. To overcome this, and at the same time ensure that the generators and cables are not overloaded with wattles current, the supply authorities often offer reduced terms to consumers whose power factor is high or impose penalties for low power factor.
Reductions in power costs can be made by taking advantage of these special terms.
Definition: The power factor of a load is defined as the ratio of active power to apparent power, i.e. KW:KVA and is referred to as cos φ. The closer cos φ is to unity, the less reactive power is drawn from the supply.
Why PFI is Necessary:
* A reduction in the overall cost of electricity can be achieved by improving the power factor to a more economic level.
* The supply will be able to support additional load which may be of benefit for an expanding company.
* Reducing the load on distribution network components by power factor improvement will result in an extension of their useful life.
Application: Any installation, including the following types of machinery or equipment is likely to have a low power factor, which can be corrected incorporating a suitable PFI plant, with a consequent saving in charges:
a) Induction Motor of all types.
b) Power Thyristor installations for DC motor control & electro-chemical processes.
c) Power transformer and voltage regulators.
d) Welding machines.
e) Electric-arc and induction furnaces.
f) Choke Coils and Magnetic systems.
g) Neon signs and fluorescent lighting.
The Principles of Power Factor Correction:
Under normal operating conditions certain electrical loads (e.g. induction motors, welding equipment, are furnaces and fluorescent lighting) draw not only active power from the supply, but also inductive reactive power (KVAr). This reactive power is necessary for the equipment to operate correctly but could be interpreted as an undesirable burden on the supply.
If cosφ =1 the transmission of 500KW in a 400V three phase mains requires a current of 722 A. The transmission of the same effective power at a cosφ = 0.6 would require a far higher current, namely 1203A. Accordingly, distribution and transmission equipment as well as feeding transformers have to be dimensioned for this higher load. Further, their useful life may decrease.
* For systems with a low power factor the transmission of electric power in accordance with existing standards results in higher expenses both for the supply distribution companies and the consumer.
Another reason for higher expenses are losses incurred via heat dissipation in the leads caused by the overall current of the system as well as via the windings of both transformers and generators.
If we assume for our above example that with cosφ = 1 the power dissipated would amount to about 10kw, then a power factor of 0.6 would result in a 180% increase in the overall dissipation i.e. 28kw.
* In general terms, as the power factor of a three phase system decrease, the current rises. The heat dissipation in the system rises proportionately by a factor equivalent to the square of the current rise.
This is the main reason behind why electricity supply companies in modern economies demand reduction of the reactive load in their networks via improvement of the power factor. In most cases, special reactive current tariffs penalize consumers for poor factor.
Methods of Power Factor Correction:
Opposing capacitive reactive power resulting from the connection of a correctly sized capacitor can compensate for the inductive reactive power required by the electrical load. This ensures a reduction in the reactive power drawn from the supply and is called Power Factor Correction.
Most common methods of power factor correction are:
Single or fixed PFC, compensating for the reactive power of individual inductive loads right on the spot and reducing the load in the feeding leads (typical for single, permanently operated loads with constant and/or big power).
Group PFC– connecting one fixed capacitor to a group of simultaneously operated inductive loads (e.g. group of motors, discharge lamps).
Central PFC, typical for large electrical systems with fluctuating load where it is common to connect a number of capacitors to a main power distribution station or substation. The capacitors are controlled by a microprocessor based relay which continuously monitors the reactive power demand on the supply. The relay connects or disconnects the capacitors to compensate for the actual reactive power of the total load and to reduce the overall demand on the supply.
A typical power factor correction system would incorporate a number of capacitor section determined by the characteristics and the reactive power requirements of the installation under consideration.
Sections of 12.5 KVAr, 25 KVAr, and 50 KVAr are usually employed. Larger stages (e.g. 100 KVAr and above) are achieved by cascading a number of smaller sections. This has the beneficial effect of reducing fluctuations in the mains caused by the inrush currents to the capacitors and minimizes supply disturbances. Where harmonic distortion is of concern, appropriate systems are supplied incorporating detuning reactors.
Major Components:
Capacitor Bank
PFC Relay
Magnetic Contactor
Bus Bar
Panel
General Description:
The power factor regulator combines comprehensive operation with user-friendly control setting. It uses numerical techniques in computing the phase difference between the fundamentals of current and voltage, thus precise power factor measurement is achieved in presence of harmonics.
The power factor regulator is designed to optimize the control of reactive power compensation. Reactive power compensation is achieved by measuring continuously the reactive power of the system and then compensated by the switching of capacitor banks. The sensitivity setting optimizes the switching speed. With the built-in intelligent automatic switching program, the power factor regulator further improves the switching efficiency by reducing the number of switching operations required to achieve the desired power factor.
Usage of the capacitor bank is evenly distributed by the intelligent switching algorithm. This ensures uniform ageing of the capacitors and the contactors used.
The four-quadrant operation feature allows the power factor regulator to operate correctly in the case of active power feed back to the mains where regenerative power sources used.
Harmonic current in the system can be harmful to the capacitor bank. This power factor regulator is capable of measuring the total harmonic distortion (THD) in the system and produce an alarm if the THD level is higher than the pre-set value. Other alarms include under/over compensate alarm, under/over current alarm and under/over voltage alarm.
Current Transformer (CT) polarity is important in determining the correct phase angle different between the current and voltage hence the power factor. This power factor regulator will automatically correct the CT polarity internally in the event that the polarity is reversed.